Welcome to the Wendy Thomas Laboratory Home Page
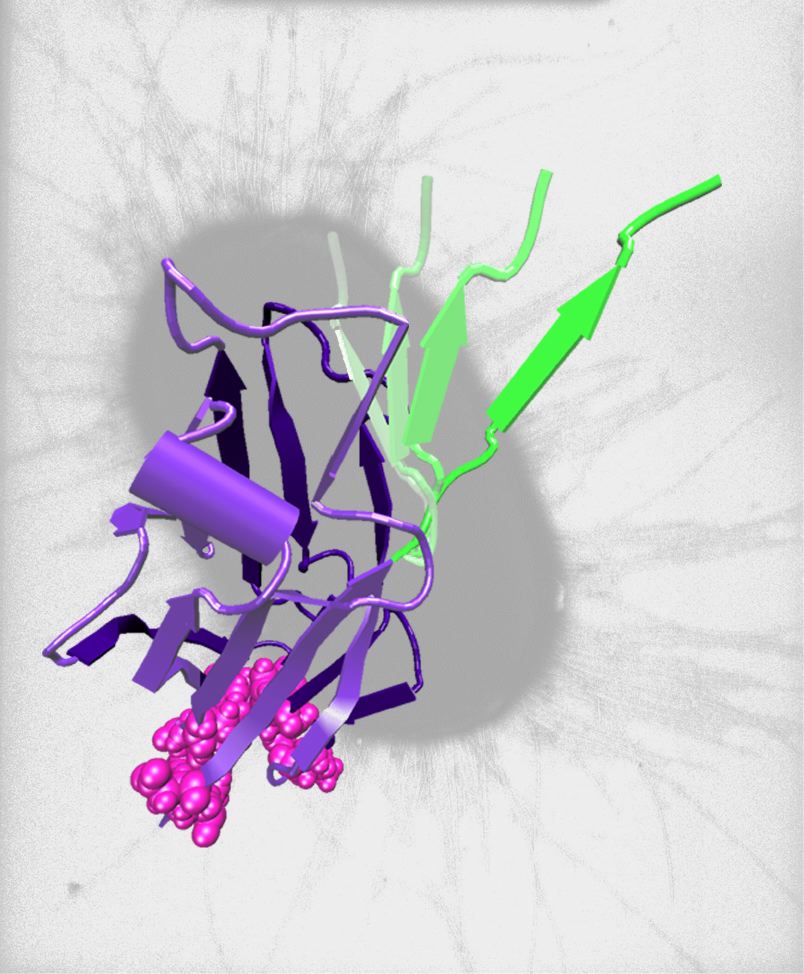
Our laboratory studies the regulation of adhesive proteins. Our work includes both basic science and translational work in engineered materials. While we study protein allostery in general, we are particularly interested in adhesion proteins that are regulated by mechanical forces that are exerted from external sources such as fluid flow or from internal sources such as cytoskeletal tension between cellular adhesion sites. We study the interface between mechanics and chemistry to understand how these mechanical forces regulate molecular structure and function.
Much of our work has been on "catch bonds" that are activated rather than torn apart by tensile mechanical force. In particular, we study mechanical regulation of bacterial biofilms (important in infectious diseases) and of thrombosis (critical in both bleeding disorders and heart attacks and strokes). Finally, we are engineering bio-inspired "smart" adhesives that are regulated by mechanical force, for medical microrobotics and drug delivery.
Research in the Thomas Lab is interdisiciplinary, with students from many departments including Bioengineering, Biomolecular Structure and Design, Biochemistry, Microbiology,and Mechanical Engineering. We integrate computational and experimental tools to address our questions. Some lab members do solely experimental work or solely computational work, while others do both.
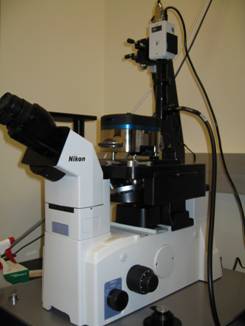
We use atomic force microscopy and microfluidic devices to apply forces to single adhesive bonds or molecules as well as to cells, communities of cells, and adhesive materials.
We seek to understand the structural basis of observed behavior by using computational protein structure tools (Molecular dynamics and Rosetta) that provide hypotheses about structure-function relationships at atomic-level resolution.
We design proteins with modified function in order to test our structure-functon hypotheses and also to develop proteins for new technologies. We use site-directed mutagenesis and other molecular biology tools to make proteins. We use both computational tools and selection methods (diversity libraries and directed evolution) to design the proteins.
Finally, we write our own software to carry out meso-scale stochastic mechanics simulations that bridge the molecular behaviors we study to the cell and larger-scale functions, to address questions about physiology and disease and to develop engineered materials.
We hope to apply our knowledge of the mechanical properties of biological adhesion to engineer novel materials and to design therapeutic interventions.
1) TRANSLATIONAL ENGINEERING AND BIOMATERIALS: We are engineering bio-inspired smart adhesives. In our "BioCatch" project, we design mechanically regulated adhesives that may be used for medical micro-robotics and drug delivery. In our "Actibodies" project, we design activatable anti-body like proteins that bind to targets of interest, but can be triggered to detach. This should allow improved control in drug delivery, biosensing, self-assembly, and separation technologies. We always welcome new collaborators who see potential applications for our technology.
2) INFECTION AND BIOFILMS: Bacteria adhere to tissues and biomaterials to form biofilms that allow them to resist antibiotics and immune responses. We study how this binding is regulated, particular by mechanical force, since we have determined that most bacterial adhesion we have tested is actually activated by force and fluid flow. Understanding how bacteria regulate adhesion should lead to development of novel classes of "anti-adhesive" antibiotics that leave bacteria vulnerable to other means of eradication by preventing biofilm formation.
3) CARDIOVASCULAR RESEARCH: We are also getting a better understanding of how high fluid flow activates platelet adhesion and aggregation. This high shear mechanism of thrombosis can stop bleeding in injured arteries but can also completely block an artery that is narrowed due to atherosclerosis, and thus cause a heart attack or stroke. We hope that by understanding how mechanical force induces thrombosis, we can specifically prevent heart attacks and strokes with minimal risk of bleeding disorders.
Biomaterials & Tissue Engineering: We design novel biomaterials in the form of adhesive coatings that are reversible and nonfouling. We also study two major issues for biomaterial implants: bacterial infection and biofilms and thrombosis. Prof. Thomas is associated with UWEB.
Molecular Bioengineering: We use a variety of nanotechnology tools to learn how proteins are regulated by mechanical force, antibodies, and other means. We then design or redesign these proteins for technological applications such as developing nanostructured adhesives with novel adhesive properties, and for designing new ways to prevent infection or thrombosis. Prof. Thomas is a member of the UW Center for Nanotechnology , the Molecular and Cellular Biology PhD Program, and the UW Molecular Biophysics Training Grant.
Integrative Physiology, Systems Biology, Synthetic Biology: We develop meso-scale simulation tools for understanding cellular adhesive processes at the systems level, and for designing nanostructured adhesives.
Understanding Nature Through Engineering. We seek to understand many areas of biomechanics, including mechanotransduction and cell adhesion, with an emphasis on platelets (involved in thrombotic and bleeding disorders) and bacteria (both commensal and infectious).