Nicaraguan
Campesino Coffee Roasted Under Different Conditions and Analyzed Using Gas
Chromatography
and Principal Component Analysis
By Spencer Ubben,* Susan C.
Jackels,* and Charles F. Jackels**
*Chemistry Department,
Seattle University, Seattle, Washington
**Computing and Software Systems and Science and Technology Programs,
University of Washington Bothell, Bothell, Washington
RESULTS
As heating element power levels
decreased, the time of roast
increased with 60% and 70% having been affected the greatest.
-
-
-
-
-
-
-
-
-
-
-
-
-
-
-
Figure 2. Temperature
versus Time plots for coffee roasted
with various percents of heating element power.
Figure 3. Key aroma components with characteristics and origin from roasting (3)(5).
Figure 4. Relative change in Peak Area % as a function of roast level for key aroma components.
Figure 5. Roasted coffee training set loadings of PC 1 versus GC retention time in seconds.
The largest increase of
nitrogen-containing heterocyclic compounds
such as pyridine was found in the 60% roast as seen on figure 4.
The increasing concentration levels seen in pyridine, 2-Furanmethanol, 2-Furanmethanol, acetate and decreasing concentration of 2-Furancarboxaldehyde, 5-methyl- and 2-Propanone, 1-(acetyloxy)- are confirmed with three decreasing peaks anti correlated with two increasing peaks as seen on figure 5.
ABSTRACT
The overall research goal is to develop an analytical
method based on gas
chromatography of roasted coffee aroma in order to correlate aroma components with quality, origin and distinctiveness of
various coffees. In this initial phase of the research, we took one coffee,
Nicaraguan Campesino green coffee,
and roasted it varying the roast power from 100% to 60% in order to create roasted samples of different character (mild to
dark roast). The ground, roasted coffee was head-space sampled by solid phase
micro-extraction and the aroma
components separated and detected by GC-FID.
Principal component
analysis was applied to the data sets, resulting in four components responsible for over 80% of the variation. PC1 (67.3% of variance) differentiated the 60% roast from the other roasts and
identified 6 GC peaks as responsible
for the variation. GC-MS was used to
identify the compounds in the peaks. In conclusion, GC analysis of roasted coffee
aroma can differentiate coffees
of different roast conditions.
INTRODUCTION
With
coffee prices reaching the lowest inflation-adjusted prices in 100 years in 2001, the impact remains
considerable among the
poverty-stricken coffee producers
in developing countries (1). Nicaragua, the second poorest country in the Western hemisphere, has lost roughly 122,000 jobs to the crisis and has had a notable impact on family farms (2). One solution to the crisis is to support family farms with development programs.
Cooperative groups of farmers are working toward organic and fair-trade certifications and are
using inexpensive methods to
farm and produce better coffee for
specialty markets in developed countries.
Coffee farmers will benefit from
feed back on the characteristics
and quality of their coffee. Traditional coffee tasting is expensive, subjective and difficult to
obtain. Therefore, we are interested in developing
an analytical method based
on gas chromatography of roasted
coffee aroma.
Figure 1. Gas Chromatogram of aroma compounds in roasted Campesino coffee.
Over
1000 volatile chemicals have been identified in brewed coffee as contributing to the smell and
taste. Gas chromatography can separate the compounds for further analysis. The volatile chemicals in extracts
from roasted coffee beans
were analyzed by gas chromatography
in a recent study by Moon, et al, who found that furfural derivatives and furanones were yielded in relatively high concentrations under mild roasting conditions and then decreased at higher roasting intensities (3).
SPME (solid phase micro extraction)
has been applied to the study of
coffee brew aroma as a simple, rapid, solvent-free, and inexpensive method (4). Of the available fibers for SPME, such as polydimethylsiloxane
METHODS
-Campesino coffee from
Nicaragua purchased from Café Campesino,
Inc., Americus, Georgia.
-250 grams of green
coffee was roasted in a Hottop Coffee
Roaster with the % power level remaining constant at 60, 70, 80, 90, 100 or Auto setting.
-After roasting, the
coffee was stored in a sealed container
at room temperature to allow the roast to develop for two days.
-Coffee was then ground
to a fine powder at setting 5 in a
Rancillo Rocky grinder.
-3.5 grams were placed
in a 35 mL headspace vial and maintained
in the oven at 60 degrees for 30 minutes and then sampled for 5 minutes with a SPME
( DVB/CAR/PDMS).
-SPME was placed in the
GC injection port and the sample
was run. Injection was in the splitless mode, initial temperature 40 o C; temperature
ramp rate: 3 oC per minute to 190 o C. Temperature
held at 190 for 10 min.
Temperature ramp rate: 15 o C per minute 190 to 250 o C. Total run time was 66 minutes.
-Data was scaled
normalized and aligned using “COW” (Tomasi
C., van den Berg F., and Andersson C., 2004).
-
PCA was performed with PLS Toolbox 5.5/MATLAB.
CONCLUSIONS
The results indicated that it is
possible to classify the aroma
profile of roasted coffee by roast level.
With longer
roasts such as 60% and 70% bringing about the largest decrease in concentration levels of the sweet, bread-like and caramellic furans and largest increase of
pleasant-burnt/smoky
nitrogen-containing heterocyclic compound
such as pyridines, pyrazines and pyrroles (3).
This knowledge can be applied to
future research aimed at
coffee quality improvement. Results
from this and future research may
lead to new, inexpensive techniques
that can be utilized and applied by impoverished
coffee farmers in Nicaragua and other poverty-stricken countries impacted by the coffee
crisis.
LITERATURE
CITED
1. Oxfam International. 2003. Mugged:
Poverty in your Coffee Cup. Oxford, U.K.: Oxfam Publishing Nov. 2003. 58P.
2.
International Coffee Organization. 2003. Impact of the coffee crisis on poverty in producing
countries. London:
International Coffee
Organization. International Coffee
Council document 89-5. 10 p. Available:
http://www.ico.org/documents/icc89-5r1e.pdf.
3.
Moon, J. K.; Shibamoto, T. Role of Roasting Conditions in the Profile
of Volatile Flavor Chemicals Formed from Coffee Beans. J. Agric. Food Chem. 2009, 57,
5823-5831.
4.
Lopez-Galilea, I.; Fournier, N.; Cid, C.; Guichard, E. Changes in
Headspace Volatile Concetrations of Coffee Brews Caused by the Roasting Process and the Brewing
Procedure. J. Agric. Food Chem. 2006, 54, 8560-8566.
5. Gonzalez-Rios O., et al. Impact of “ecological” post-harvest
processing on coffee aroma: II. Roasted coffee. Journal of Food Composition and Analysis. 2007, 20,
297-307.
Background Image from Flickr:
http://flickr.com/photos/tonx/145685020/
STATEMENT
OF PURPOSE
The purpose is to use the coffee
aroma profile of freshly roasted
coffee to develop a method involving gas chromatographic separation and subsequent PCA. Our goal is to characterize and differentiate the aroma components under a range of roast conditions for a single coffee.
(PDMS), divinylbenzeze(DVB), or
carboxen (CAR), a SPME fiber
with all three adsorbents coated on it works best for adsorbing coffee aroma (4).
In order to classify and make use of
hundreds of different
brewed coffee compounds, principal component analysis (PCA) was used. Principal component analysis has been used in the past for analysis and
classification of a
multitude of compounds. In a recent
study which used PCA to
differentiate coffee brew samples, it was found that conventional and torrefacto roasted coffee brews were successfully classified by use of PCA
(4).
This study was conducted in order to
characterize coffee by
use of PCA on aroma profiles of coffee that had been roasted under varying levels of roast
power. Aroma was sampled over freshly ground coffee. If PCA is successful in differentiating one coffee roast from another based solely on the gas chromatography data, then it may be possible to apply the same technique to differentiate coffees from different regions as well as various growing conditions.
Figure 6.
Roasted coffee scores of training set verses validation set.
Note: 60% coffee roasts were
successfully differentiated from other roasts using previously constructed training set. Data from a different technician, SPME fiber, coffee batch and 6 months later were able to
be classified using the training set, thus demonstrating robustness of
method.
Figure 7. Roasted coffee scores for PC1 versus PC
2.
- Principle component 1 is
responsible for 67.45% of the variance
in the roasted coffee samples while PC 2 has a very little impact at only 9.47%. As seen in figure 7 the 60% roasts are classifiable by PCA . 70% roasts are also brought out but not to the degree of 60% roast.
ACKNOWLEDGEMENTS
The authors would like to thank their
respective institutions, Seattle University and University of Washington Bothell, for the resources needed to accomplish this
project.
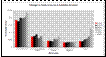